Material and device properties modification by electrochemical charge injection in the absence of contacting electrolyte for either local spatial or final states
a charge injection and electrochemical technology, applied in the direction of crystal growth process, disinfection, other medical devices, etc., can solve the problems of limiting the applicability of devices, limiting the application of materials, and providing problematic structural changes
- Summary
- Abstract
- Description
- Claims
- Application Information
AI Technical Summary
Benefits of technology
Problems solved by technology
Method used
Image
Examples
example 1
[0218] This example shows that the electrical conductivity of carbon nanotube electrode sheets can be continuously varied by about an order of magnitude using predominately non-faradaic electrochemical charge injection in 1 M NaCl electrolyte
[0219] These nanotube sheets are fabricated analogously to a previously described method (A. G. Rinzler et al., Appl. Phys. A 67, 29 (1998)) by dispersing the carbon nanotubes in surfactant-containing aqueous solution and filtering this nanotube dispersion though through a 47 mm diameter poly(tetrafluoroethylene) filter sheet (Millipore LS) under house vacuum. A sheet of nanotubes collected on the filter paper, which was washed using water and methanol, dried, and then lifted from the filter paper substrate to provide a free-standing SWNT sheet. The density of these nanotube sheets is about 0.3 g / cm3, versus the density of about 1.3 g / cm3 for densely packed nanotubes having close to the observed average nanotube diameter. Hence the void volume ...
example 2
[0224] This example demonstrates that tuning the electrical conductivity of single-wall nanotube sheets over an order-of-magnitude range can also be accomplished using an organic electrolyte, instead of the aqueous electrolyte of Example 1.
[0225] The experimental procedure was exactly the same as for Example 1, except that the 1M NaCl aqueous electrolyte was replaced with either 0.1M TBAPF6 (tetrabutyl ammonium hexafluorophosphate) or 0.1M TBABF4 (tetrabutyl ammonium tetrafluoroborate) dissolved in acetonitrile and the Ag / AgCl reference electrode was replaced with the Ag / Ag+ reference electrode. There were no significant differences noted between charge-injection tuning of the nanotube sheet conductivity in 0.1 M TBAPF6 / acetonitrile and in 0.1 M TBABF4 / acetonitrile.
[0226] Because the potential window of non-faradaic reaction in non-aqueous electrolyte is much wider than for aqueous electrolytes, the potential can be changed up to +1.0V (versus Ag / Ag+) in the positive potential dir...
example 3
[0229] This example shows that the hysteresis in properties tuning can be significantly reduced if electrical conductivity is varied by charging the amount of injected charge, as opposed to being controlled by changing applied voltage. This point is illustrated for the 0.1M tetrabutylammonium hexafluorophosphate / acetonitrile electrolyte by comparing the hysteresis in the electrical conductivity versus potential (FIG. 1 and Example 1) with those in FIG. 2, where electrical conductivity is plotted versus charge per carbon in the nanotube working electrode. The decrease that is obtained in hysteresis in going from voltage control of conductivity to charge control is even larger for the aqueous 1 M NaCl electrolyte of Example 1. The data in FIG. 2 also shows the dependence of four-point electrical conductivity upon the amount of injected charge (per carbon) for the nanotube sheet is nearly identical for the 1 M NaCl electrolyte of Example 1 and the 0.1M tetrabutylammonium hexafluorophos...
PUM
Property | Measurement | Unit |
---|---|---|
Transparency | aaaaa | aaaaa |
Biocompatibility | aaaaa | aaaaa |
Radioactivity | aaaaa | aaaaa |
Abstract
Description
Claims
Application Information
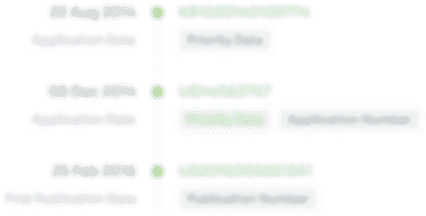
- Generate Ideas
- Intellectual Property
- Life Sciences
- Materials
- Tech Scout
- Unparalleled Data Quality
- Higher Quality Content
- 60% Fewer Hallucinations
Browse by: Latest US Patents, China's latest patents, Technical Efficacy Thesaurus, Application Domain, Technology Topic, Popular Technical Reports.
© 2025 PatSnap. All rights reserved.Legal|Privacy policy|Modern Slavery Act Transparency Statement|Sitemap|About US| Contact US: help@patsnap.com