System having unmodulated flux locked loop for measuring magnetic fields
a technology of magnetic field and flux lock, which is applied in the direction of magnetic measurement, instruments, measurement devices, etc., can solve the problems of small limiting field strength providing little practical value, distortion-producing, non-linear, bulky, and achieves fewer, smaller and less expensive electronic components. , the effect of eliminating distortion-producing, non-linear, bulky and expensive rf components
- Summary
- Abstract
- Description
- Claims
- Application Information
AI Technical Summary
Benefits of technology
Problems solved by technology
Method used
Image
Examples
Embodiment Construction
, below.
BRIEF DESCRIPTION OF THE DRAWINGS
[0022] A preferred embodiment of the present invention is described in detail below with reference to the attached drawing figures, wherein:
[0023]FIG. 1 is a block diagram showing a preferred embodiment of the system of the present invention, comprising a direct-feedback FLL connected by unbalanced RF coaxial transmission lines to a SQUID;
[0024]FIG. 2 is a first circuit schematic of a first integrator network component of the direct-feedback FLL of FIG. 1;
[0025]FIG. 3 is a first equation describing the circuit schematic of FIG. 2;
[0026]FIG. 4 is a second circuit schematic of a second integrator network component of the direct-feedback FLL of FIG. 1; and
[0027]FIG. 5 is a second equation describing the second circuit schematic of FIG. 4.
DETAILED DESCRIPTION OF A PREFERRED EMBODIMENT
[0028] With reference to the figures, a system 10 for measuring magnetic fields is herein described, shown, and otherwise disclosed in accordance with a prefe...
PUM
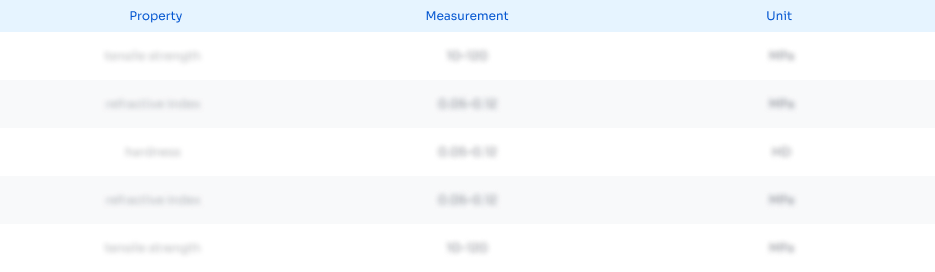
Abstract
Description
Claims
Application Information
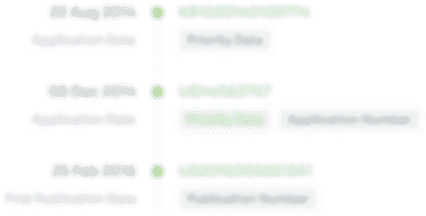
- R&D Engineer
- R&D Manager
- IP Professional
- Industry Leading Data Capabilities
- Powerful AI technology
- Patent DNA Extraction
Browse by: Latest US Patents, China's latest patents, Technical Efficacy Thesaurus, Application Domain, Technology Topic, Popular Technical Reports.
© 2024 PatSnap. All rights reserved.Legal|Privacy policy|Modern Slavery Act Transparency Statement|Sitemap|About US| Contact US: help@patsnap.com