Using Additive Manufacturing For Turbine Engines To Improve Performance
Technology Background and Goals
Additive manufacturing offers several advantages over traditional manufacturing methods, including the ability to create complex geometries, reduce material waste, and enable lightweight designs. By leveraging these capabilities, turbine engine components can be optimized for improved aerodynamic performance, increased fuel efficiency, and reduced emissions. Additionally, additive manufacturing allows for the integration of multiple parts into a single component, simplifying assembly and reducing the overall weight of the engine.
Market Demand Analysis
- Aerospace Industry Demand
Additive manufacturing (AM) has significant potential in the aerospace industry, particularly for turbine engine components. The demand is driven by the need for lightweight, high-performance parts with complex geometries that are difficult to produce using traditional manufacturing methods. - Cost Reduction
AM can reduce manufacturing costs by minimizing material waste, enabling on-demand production, and reducing the need for tooling and inventory. This cost-effectiveness is particularly attractive for low-volume, high-value components. - Design Flexibility
AM allows for greater design freedom, enabling the creation of intricate geometries and internal features that are challenging or impossible to achieve with conventional manufacturing techniques. This flexibility can lead to improved performance and efficiency. - Repair and Maintenance
AM can be used for repair and maintenance applications, such as producing replacement parts on-demand, reducing downtime and extending the lifespan of turbine engines. - Customization and Personalization
AM enables the production of customized and personalized components, catering to specific requirements or individual needs. This can be advantageous in niche markets or for specialized applications.
Technology Status and Challenges
- Material Limitations
Current additive manufacturing (AM) materials have limited mechanical properties, such as strength, ductility, and fatigue resistance, compared to traditional manufacturing methods. This poses challenges for high-performance applications like turbine engines. - Process Inconsistencies
AM processes can suffer from inconsistencies in part quality due to factors like powder quality, environmental conditions, and process parameter variations. This can lead to defects and performance issues in critical components. - Design Constraints
The design freedom offered by AM is still limited by factors like support structure requirements, part orientation, and post-processing needs. These constraints can restrict the full potential of AM for complex geometries. - Qualification and Certification
Establishing robust qualification and certification processes for AM-produced parts, especially for safety-critical applications like turbine engines, remains a significant challenge due to the complexity and variability of AM processes. - Cost and Scalability
While AM offers advantages in customization and design flexibility, the high costs of materials, equipment, and post-processing can limit its widespread adoption, especially for large-scale production.
Technology Evolution Path
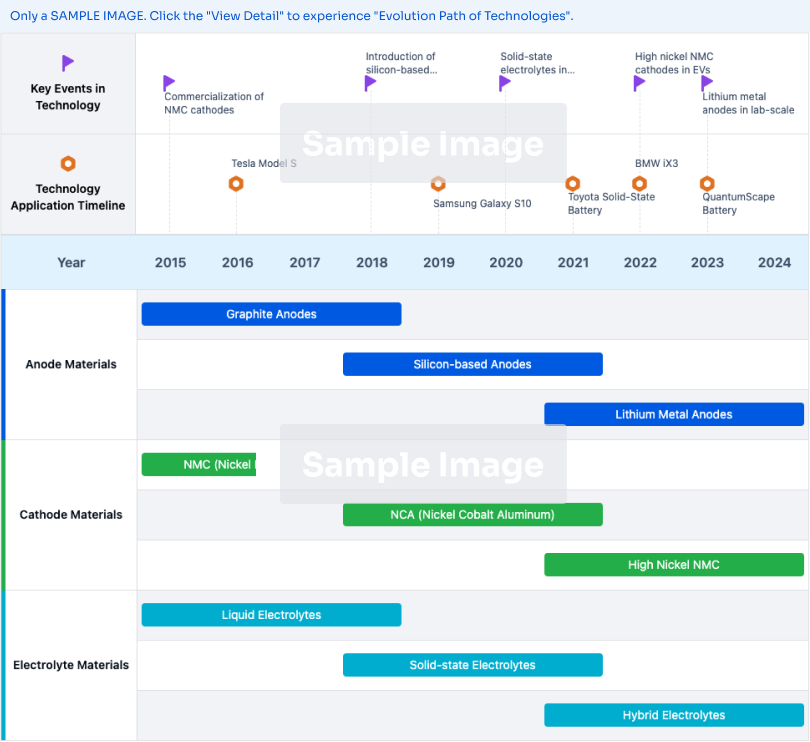
Current Technical Solutions
01 Turbine engine performance optimization
Various techniques for optimizing turbine engine performance, such as adjusting operating parameters, modifying component designs, or implementing control systems to improve efficiency, reduce emissions, or enhance overall performance.- Turbine engine performance optimization: Techniques for optimizing turbine engine performance, including adjusting operating parameters, modifying component designs, and implementing control systems to improve efficiency, reduce emissions, and enhance overall performance.
- Turbine blade design and cooling: Improved turbine blade designs and cooling mechanisms to increase durability, efficiency, and allow higher operating temperatures for better engine performance.
- Combustion system optimization: Optimizing combustion systems through modifications to combustor design, fuel injection systems, and advanced combustion control strategies for improved performance, reduced emissions, and increased efficiency.
- Turbine engine control systems: Advanced control systems with sensors, algorithms, and actuators to monitor and adjust engine parameters in real-time for optimal operation under varying conditions.
- Turbine engine component design and materials: Advancements in materials and component designs for compressors, combustors, and turbines to improve durability, efficiency, and overall engine performance.
02 Turbine blade design and cooling
Improved turbine blade designs and cooling mechanisms to increase durability and efficiency, allowing for higher operating temperatures and better overall engine performance.03 Combustion system optimization
Optimizing combustion systems through modifications to combustor design, fuel injection systems, or advanced combustion control strategies for improved performance, reduced emissions, and increased fuel efficiency.04 Turbine engine control systems
Advanced control systems with sensors, algorithms, and actuators to monitor and adjust engine parameters in real-time for optimal operation under varying conditions.05 Turbine engine component design and materials
Advancements in materials science and component design for compressors, combustors, and turbines to improve durability, efficiency, and overall engine performance.
Main Players Analysis
RTX Corp.
General Electric Company
Key Technology Interpretation
- The use of additively manufactured wear surfaces, which are designed to extend the lifespan of the combustor components and reduce material degradation. this allows for more efficient and cost-effective manufacturing processes, as well as the potential for improved performance and durability of the combustor.
Potential Additive Manufacturing Innovations
- Turbine Engines with Additively Manufactured Ceramic Matrix Composites
- Turbine Engines with Additively Manufactured Hybrid Metallic-Ceramic Components
- Turbine Engines with Additively Manufactured Lattice Structures for Thermal Management
Additive Manufacturing Regulatory Landscape
In the United States, the Federal Aviation Administration (FAA) has established a roadmap for certifying AM parts for aircraft, outlining requirements for design, production, and continued airworthiness. The FAA collaborates closely with organizations like ASTM International and SAE International to develop industry-wide standards. Similarly, the European Union Aviation Safety Agency (EASA) has issued regulations and guidance material for AM in aviation, emphasizing the importance of robust quality management systems.
International organizations like the International Civil Aviation Organization (ICAO) and the International Organization for Standardization (ISO) are also playing a crucial role in harmonizing AM regulations globally. The ISO/ASTM 52900 series of standards provides a comprehensive framework for AM processes, materials, and test methods, facilitating cross-border collaboration and trade in AM components.
Additive Manufacturing Environmental Impact Assessment
The environmental impact assessment should encompass the entire AM process, from raw material extraction and processing to component manufacturing, use, and end-of-life disposal or recycling. Key factors to consider include energy consumption, greenhouse gas emissions, waste generation, and resource depletion. Additionally, the potential for material and energy savings through optimized designs and reduced waste should be evaluated.
Furthermore, the assessment should examine the environmental implications of different AM technologies, such as powder bed fusion, directed energy deposition, and material extrusion. Each technology may have unique environmental considerations, including the type of materials used, energy requirements, and waste streams generated. Comparative analyses with traditional manufacturing methods can provide valuable insights into the relative environmental impact of AM for turbine engine components.