
What Is a Standing Wave?
A standing wave is a fascinating wave phenomenon where two waves of identical frequency and amplitude travel in opposite directions, creating a stationary pattern. This interaction forms nodes—points of no movement—and antinodes, where displacement reaches its peak. Unlike traveling waves, standing waves do not transfer energy across space; instead, their oscillations remain fixed at specific positions. Commonly observed in musical instruments, vibrating strings, and resonance chambers, standing waves play a crucial role in physics, engineering, and acoustics. This article explores the principles, examples, and applications of standing wave, helping you understand their significance in everyday life and technology.
How Standing Waves Form: Interference and Reflection
Standing waves form when a wave reflects back upon itself, often at boundaries like the ends of a resonating cavity or between different media. The reflected wave interferes with the incoming wave, creating a stationary pattern where certain points remain fixed while others oscillate.
Nodes and Antinodes
Nodes are fixed points in a standing wave where the amplitude is always zero due to destructive interference. In contrast, antinodes are points of maximum displacement, where constructive interference occurs. These nodes and antinodes remain stationary, creating the characteristic standing wave pattern.
Frequency and Wavelength
The frequency of a standing wave matches the frequency of the original waves that combine to form it. The wavelength depends on the boundary conditions and the dimensions of the resonating system, determining the specific pattern of nodes and antinodes.
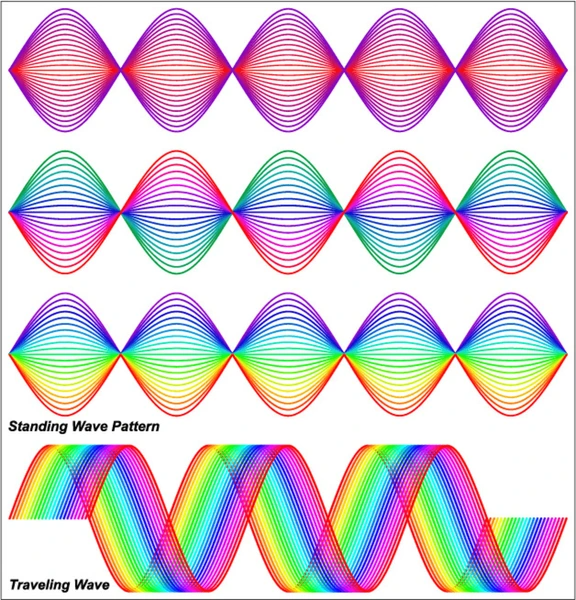
Standing Waves vs Traveling Waves
Definition and Characteristics
- Standing Waves
Standing waves form when two waves with the same frequency and amplitude move in opposite directions through a medium and interfere. This interference creates fixed points of maximum and minimum amplitude, known as antinodes and nodes, respectively. Unlike traveling waves, standing waves oscillate in place rather than moving through the medium. - Traveling Waves
Traveling waves move through a medium, transferring energy from one location to another in a specific direction. These waves are commonly observed in systems like electromagnetic waves in free space or sound waves in air.
Mathematical Representation
- Standing Waves
A standing wave is the result of two traveling waves of equal amplitude and frequency moving in opposite directions. This superposition creates nodes where the waves cancel each other and antinodes where they constructively interfere, forming a stable oscillatory pattern. - Traveling Waves
Traveling waves are described by equations that show how they propagate through space over time. These equations often involve sinusoidal functions that represent the wave’s motion and energy transfer.
Applications and Implications
- Standing Waves
Standing waves are common in systems with fixed boundaries, such as strings fixed at both ends. They are essential for understanding resonance, a phenomenon critical in acoustics, quantum mechanics, and the design of musical instruments. - Traveling Waves
Traveling waves appear in systems where waves can move freely, such as light in space or sound in open air. They are crucial for applications like communication systems, signal processing, and wave propagation studies.
Key Differences
The primary distinction between these wave types lies in their motion and energy transfer. Standing waves do not move through space; instead, they oscillate in a fixed pattern. In contrast, traveling waves propagate through the medium, carrying energy from one point to another.
Additionally, traveling waves interacting with boundaries or obstacles often create standing waves, demonstrating the dynamic interplay between these two wave types.
Applications of Standing Waves in Physics and Engineering
Telecommunications and Antenna Design
Resonating waves are essential in antenna design to improve radiation efficiency and isolate communication systems. For instance, in loop antennas, these waves ensure efficient radiation and maintain isolation across power feeders.
Liquid Crystal Displays and Optical Devices
In liquid crystal cells, resonant wave patterns influence molecular alignment, forming tunable defect arrays. These arrays act as optical vortex inducers or micro-lens arrays, adapting to a wide range of frequencies for advanced optical uses.
Volcanology and Geophysical Studies
Resonant patterns in high-speed lava channels reveal key properties of lava flows, such as viscosity and discharge rates. This data is vital for assessing volcanic hazards and understanding eruption behavior near vent areas.
Acoustic and Ultrasonic Applications
Resonating acoustic patterns enhance engineering processes like particle separation. By reducing fluid velocity inconsistencies, they maximize separation efficiency, benefiting applications in material processing and filtration.
Medical and Biological Applications
In healthcare, resonant wave patterns in tissues enable precise applications like tissue ablation, drug delivery, and diagnostics. Using amplitude- and frequency-modulated signals ensures accurate targeting for better outcomes.
Water Purification and Environmental Engineering
Purification systems utilize resonant patterns to separate contaminants from water effectively. Adjusting wave frequency and intensity allows for efficient removal of solids, supporting industrial and environmental needs.
Fluid Dynamics and Pump Design
Resonating wave energy in fluid pumps enhances performance by applying pressure to the fluid. This reduces the need for mechanical components, cuts energy loss, and lowers operational costs.
Application Cases
Product/Project | Technical Outcomes | Application Scenarios |
---|---|---|
Liquid Crystal Cells Sungkyunkwan University | Standing waves manipulate molecular orientation to form tunable periodic defect arrays with concentric director profiles, serving as optical vortex array inducers or tunable micro-liquid crystal lens arrays. They exhibit unique features like diagonal nodal lines, self-adaptation to broad resonance frequencies, and step-like wavelength increase with increasing frequency. | Optics, displays, and singular optics applications. |
Lava Flow Monitoring Simon Fraser University | Standing waves in high-speed lava channels are used to estimate rheological properties of lava flows, enabling the determination of important eruption parameters like discharge rate and apparent viscosity. | Volcanology and geophysical studies for understanding lava emplacement and hazard assessment. |
Acoustophoretic Bioreactors FloDesign Sonics, Inc. | Multi-dimensional acoustic standing waves are used to retain cells and separate biomolecules, enabling efficient and cost-effective biomolecule harvesting with reduced downtime and operational complexity. | Bioreactor applications for continuous and efficient filtration and separation of biomolecules. |
Wastewater Purification System Korea Institute of Construction Technology | Standing waves are used to gather contaminants in wastewater, enabling efficient sedimentation and separation of solid materials from contaminated water. | Water treatment and purification applications for removing solid contaminants from wastewater. |
Bitumen Recovery System Suncor Energy, Inc. | Standing waves are induced in bitumen reserves at resonant frequencies of the formation rock, facilitating bitumen recovery by enhancing the extraction process. | Oil and gas industry for enhanced bitumen recovery from bitumen reserves. |
To get detailed scientific explanations of standing waves, try Patsnap Eureka.
