
What is Nitinol?
Nitinol, an intermetallic compound of nickel and titanium, is a unique shape memory alloy with remarkable properties, including superelasticity, shape memory effect, biocompatibility, and corrosion resistance. These properties make it an ideal material for various applications, particularly in the medical field.
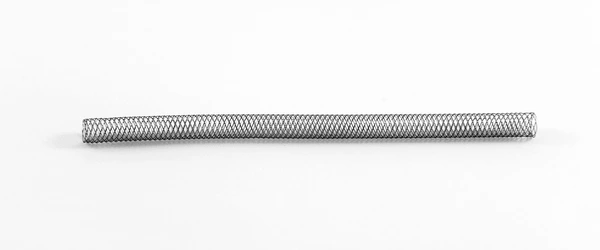
Mechanism of Nitinol
Phase Transformation and Crystal Structures
At high temperatures, it assumes an interpenetrating simple cubic crystal structure known as austenite (parent phase). Upon cooling below the transformation temperature, it spontaneously transforms to a more complicated monoclinic crystal structure called martensite. This martensitic transformation is the key mechanism behind Nitinol’s shape memory and superelastic behavior.
Shape Memory Effect
The shape memory effect allows it to undergo deformation at one temperature and recover its original, undeformed shape upon heating above the transformation temperature. 1 This is due to the reversible austenite-martensite phase change, where the martensite phase “remembers” the original austenitic shape.
Superelasticity
Superelasticity, also known as pseudoelasticity, refers to Nitinol’s ability to undergo large reversible strains (up to 8%) in the austenitic phase when subjected to stress. This stress-induced martensite (SIM) is unstable above the austenitic finish temperature (Af) and reverts back to austenite upon unloading, enabling the recoverable strains.
Tailoring Properties
The properties can be tailored through various processing techniques, including composition adjustment, alloying with other elements, heat treatment, and mechanical processing. These techniques allow controlling the transformation temperatures, ductility, and relative contributions of shape memory and superelasticity.
Manufacturing of Nitinol
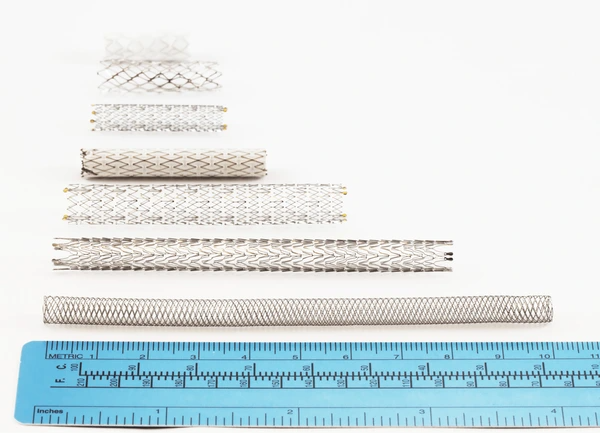
Heat Treatment
Heat treatment is a critical process for controlling the transformation temperatures and tailoring the shape memory and superelastic properties of Nitinol. The processes involve:
- Solution annealing: Heating the alloy to a high temperature (600-800°C) to homogenize the microstructure, followed by quenching to retain the austenitic phase.
- Aging: Controlled heating at lower temperatures (300-500°C) to precipitate Ni-rich phases, adjusting the transformation temperatures and enhancing the shape memory effect.
Cold Working and Annealing
Cold working (e.g., rolling, drawing) introduces dislocations and internal stresses, which can be relieved by subsequent annealing. This process can lower the austenite finish temperature (Af), enabling superelasticity at room temperature.
Additive Manufacturing
Additive manufacturing techniques, such as selective laser melting (SLM) and laser engineered net shaping (LENS), enable the fabrication of complex Nitinol structures with tailored properties. Key factors include:
- Powder quality and composition: Significantly affect the microstructure, phase transformations, and functional properties.
- Process parameters: Laser power, scan speed, hatch spacing, and cooling rates influence density, porosity, and transformation temperatures.
- Post-processing: Heat treatments are often required to optimize the microstructure and adjust the transformation temperatures.
Applications of Nitinol
Biomedical Applications
Nitinol’s biocompatibility, corrosion resistance, and unique properties have revolutionized the medical device industry. Key applications include:
- Cardiovascular devices: Self-expanding stents, heart valve components, vena cava filters.
- Orthopaedic implants: Bone staples, spinal rods, dental archwires.
- Surgical instruments: Guidewires, catheters, endoscopic tools.
The superelasticity allows minimally invasive delivery and deployment, while shape memory enables complex geometries. However, nickel release and thrombogenicity remain concerns, prompting surface modifications.
Actuators and MEMS
Nitinol’s shape memory effect enables its use as compact, solid-state actuators in various applications:
- Microelectromechanical systems (MEMS): Microvalves, micropumps, microgrippers.
- Aerospace: Deployable structures, release mechanisms, vibration dampers.
- Automotive: Temperature-responsive actuators, active engine mounts.
The large work output and high energy density of Nitinol make it advantageous over other actuator materials.
Emerging Applications
Recent research explores novel Nitinol alloys and forms for enhanced performance:
- Ternary Nitinol alloys (e.g., NiTiCr, NiTiTa) for improved biocompatibility and anti-thrombogenicity.
- Nitinol nanofibers with enhanced surface roughness for better tissue integration.
- Three-dimensional thin-film Nitinol devices for MEMS and microactuators.
These developments aim to expand Nitinol’s capabilities and open up new application areas.
Application Cases
Product/Project | Technical Outcomes | Application Scenarios |
---|---|---|
Nitinol Stents | Nitinol’s superelastic properties allow stents to be compressed for minimally invasive delivery and then self-expand to support the vessel wall, reducing the risk of restenosis compared to traditional stents. | Cardiovascular interventions, such as treating coronary artery disease and peripheral artery disease. |
Nitinol Guidewires | Nitinol’s shape memory and superelasticity enable guidewires to navigate complex vascular pathways and maintain their shape during procedures, improving procedural success rates. | Minimally invasive surgical procedures, including cardiovascular, neurological, and gastrointestinal interventions. |
Nitinol Orthodontic Archwires | Nitinol’s superelastic properties allow archwires to exert continuous, gentle forces on teeth, reducing treatment time and discomfort compared to traditional archwires. | Orthodontic treatments for correcting misaligned teeth and improving bite function. |
Nitinol Bone Staples | Nitinol’s shape memory effect allows staples to be deformed for insertion and then recover their original shape, providing a secure fit and reducing the risk of loosening or migration. | Orthopedic surgeries, such as fracture fixation and spinal fusion procedures. |
Nitinol Vena Cava Filters | Nitinol’s superelasticity allows vena cava filters to be compressed for minimally invasive delivery and then self-expand to trap blood clots, preventing pulmonary embolism. | Interventional radiology procedures for preventing life-threatening pulmonary embolism in high-risk patients. |
Latest Technical Innovations of Nitinol
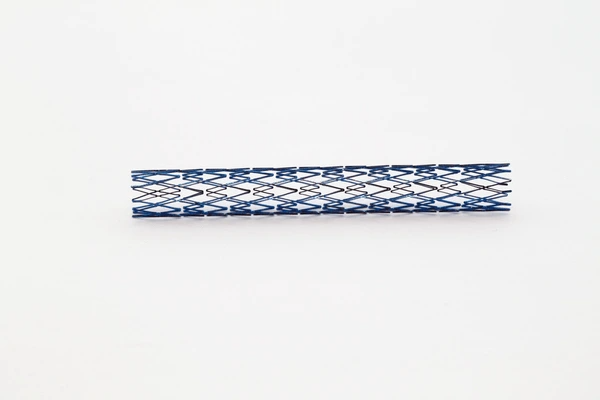
Novel Nitinol Alloy Compositions
- Ternary Nitinol Alloys: Researchers developed alloys like NiTiCr and NiTiTa by adding a third metallic element to improve nitinol’s properties and performance.
- Nickel-Free Titanium Dioxide: Researchers developed nickel-free titanium dioxide surfaces to eliminate nickel leaching risks, reducing allergic reactions in biomedical uses.
- Prealloyed Nitinol Compositions: Manufacturers produce high-hardness, corrosion-resistant Ni-36Ti to Ni-45Ti compositions using hot isostatic pressing and rolling, enhancing durability.
Processing and Manufacturing Techniques
- Magnetoelectropolishing (MEP): Researchers use MEP surface treatment to enhance the anti-thrombogenicity and biocompatibility of Nitinol implants.
- Selective Etching: Developers use selective etching processes to dissolve nickel from Nitinol’s surface layer, forming a titanium dioxide film that inhibits nickel release.
- Three-Dimensional Thin-Film Nitinol Devices: Manufacturers create thin-film Nitinol devices by depositing multiple Nitinol and sacrificial material layers on a substrate.
Phase Transformation and Property Control
- Processing Techniques: Researchers developed techniques like heat treatment, cold working, and annealing to control phase transformation temperatures and properties like superelasticity and shape memory.
- Alloying Elements: Alloying Nitinol with elements like Cu, Hf, Zr, Pt, and others modifies phase transformation temperatures and properties.
- Magnetic Field-Induced Strain: Ni-Mn-Ga alloys achieved magnetic field-induced strain of up to 10%, with operating temperatures from -40°C to 60°C
Technical Challenges of Nitinol
Novel Nitinol Alloy Compositions | Developing ternary nitinol alloys containing a third metallic element like Nickel-Titanium-Chromium (NiTiCr) and Nickel-Titanium-Tantalum (NiTiTa) to improve properties and performance. |
Nickel-free Titanium Dioxide Surfaces | Producing nickel-free titanium dioxide surfaces to eliminate potential nickel leaching and allergic reactions in biomedical applications. |
High Hardness and Corrosion Resistance | Producing high hardness, corrosion-resistant prealloyed Ni-36Ti to Ni-45Ti powder metallurgy Nitinol compositions through hot isostatic pressing and rolling. |
Three-dimensional Thin-film Devices | Manufacturing three-dimensional thin-film Nitinol devices by depositing multiple layers of Nitinol and sacrificial material on a substrate. |
Surface Treatment and Processing | Developing surface treatment and processing techniques like magnetoelectropolishing (MEP), selective etching, and three-dimensional thin-film deposition to improve biocompatibility and inhibit nickel release. |
To get detailed scientific explanations of nitinol, try Patsnap Eureka.
